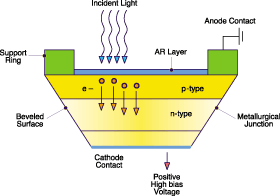
Photons entering the diode first pass through the silicon dioxide layer and then through the n and p layers before entering the depletion region where they excite free electrons and holes, which then migrate to the cathode and anode, respectively. When a semiconductor diode has a reverse voltage bias applied and the crystal junction between the p and n layers is illuminated, then a current will flow in proportion to the number of photons incident upon the junction.
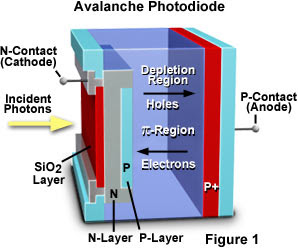
As these electrons collide with other electrons in the semiconductor material, they cause a fraction of them to become part of the photo-current - this process is called avalanche multiplication.
The gain of the APD is changed by the reverse-bias voltage applied (larger bias voltage - larger gain). However, a larger reverse-bias voltage also results in increased noise levels. Excess noise resulting from the avalanche multiplication process places a limit on the useful gain of the APD. The avalanche process introduces excess noise because every photogenerated carrier does not undergo the same multiplication. Avalanche photodiodes are capable of modest gain (500-1000), but exhibit substantial dark current, which increases markedly as the bias voltage is increased.
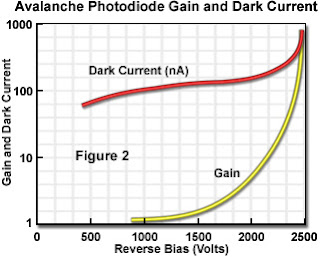
Overall, avalanche photodiodes are compact and immune to magnetic fields, require low currents, are difficult to overload, and have a high quantum efficiency that can reach 90 percent.
Avalance Photodiodes in Wavefront Sensors
It was reported\cite{takami2004performance} that avalanche photodiodes are used in wavefront sensors for adaptive optics.
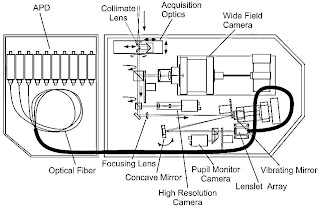
The modulated light intensity signal is sampled at each sub-aperture of a microlens array and fed through optical fibers to photon-counting avalanche photodiode (APD) modules. The current Subaru AO system has 36 sub-apertures.
A benefit of this type of sensor is that one can use photon-counting avalanche photodiodes without readout noise\cite{takami2004performance}, while for Shack-Hartmann
sensors the readout noise of CCD detector becomes the dominant error source for faint guide stars\cite{takami2004performance}. A 1000x1000 cooled CCD (EEV CCD47-20) camera covering a 20 field is used to monitor the guide star.
Another proposal\cite{aull2010adaptive} is from Lincoln Laboratory where has been investigating Geiger mode avalanche photodiode arrays integrated with CMOS readout circuits. This type of sensor counts photons digitally within the pixel, enabling data to be read out at high rates without the penalty of readout noise.
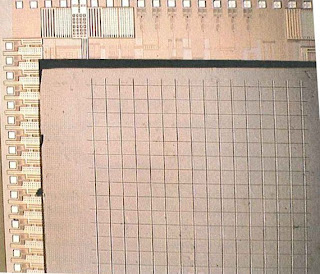
It has been demonstrated\cite{aull2010adaptive} a high-fill-factor, 16x16 quad cell APD array with monotonic centroiding response. Devices with a 32x32 format are in fabrication.
However, the dark count rate of the high-fill-factor devices is high compared with other detector technologies, and the mechanisms for this have been investigated. The dark count rate is hypothesised to arise from a combination of tunnelling current at the junction periphery, unsuppressed thermal dark current, and optical self re-triggering\cite{aull2010adaptive}.
Advances in APD for AO
Development of novel circuitry for solid state photon counting devices, based on avalanche photodiodes (PC-APD), was also reported\cite{bonaccini1994novel}. The recent development has improved silicon APDs considerably, reducing the afterpulsing effects, improving the effective Q.E., and reducing the dark current to negligible values. These new APD allow to conceive new quenching circuitry and new applications of solid state photon counters for improved adaptive optics performance\cite{bonaccini1994novel}.
The development of APDs is focusing on high bandwidth, low excess noise, and high gain bandwidth\cite{campbell2004recent}. It has been shown that lower noise and higher gain bandwidth products can be achieved by submicron scaling of the multiplication region thickness and replacing InP in the multiplication layer with Al In As\cite{campbell2004recent}. However, spatial uniformity of the photoresponse at very high gains remains work in progress.
References
\begin{thebibliography}{1}
\bibitem{takami2004performance}
H.~Takami, N.~Takato, Y.~Hayano, M.~Iye, S.~Oya, Y.~Kamata, T.~Kanzawa,
Y.~Minowa, M.~Otsubo, K.~Nakashima, et~al.
\newblock {Performance of Subaru Cassegrain adaptive optics system}.
\newblock {\em PUBLICATIONS-ASTRONOMICAL SOCIETY OF JAPAN}, 56(1):225--234,
2004.
\bibitem{aull2010adaptive}
B.F. Aull.
\newblock {Adaptive optics wavefront sensors based on photon-counting detector
arrays}.
\newblock 2010.
\bibitem{bonaccini1994novel}
D.~Bonaccini, S.D. Cova, M.~Ghioni, R.~Gheser, S.~Esposito, and
G.~Brusa-Zappellini.
\newblock {Novel avalanche photodiode for adaptive optics}.
\newblock 2201:650, 1994.
\bibitem{campbell2004recent}
J.C. Campbell, S.~Demiguel, F.~Ma, A.~Beck, X.~Guo, S.~Wang, X.~Zheng, X.~Li,
J.D. Beck, M.A. Kinch, et~al.
\newblock {Recent advances in avalanche photodiodes}.
\newblock {\em Selected Topics in Quantum Electronics, IEEE Journal of},
10(4):777--787, 2004.
\end{thebibliography}
No comments:
Post a Comment